The basic ingredient of Adenosine Triphosphate (ATP) is the nucleotide adenosine combined with three phosphate groups. ATP is often referred to as the “molecular currency” of the cell because it stores and transfers energy within cells for various biochemical processes.
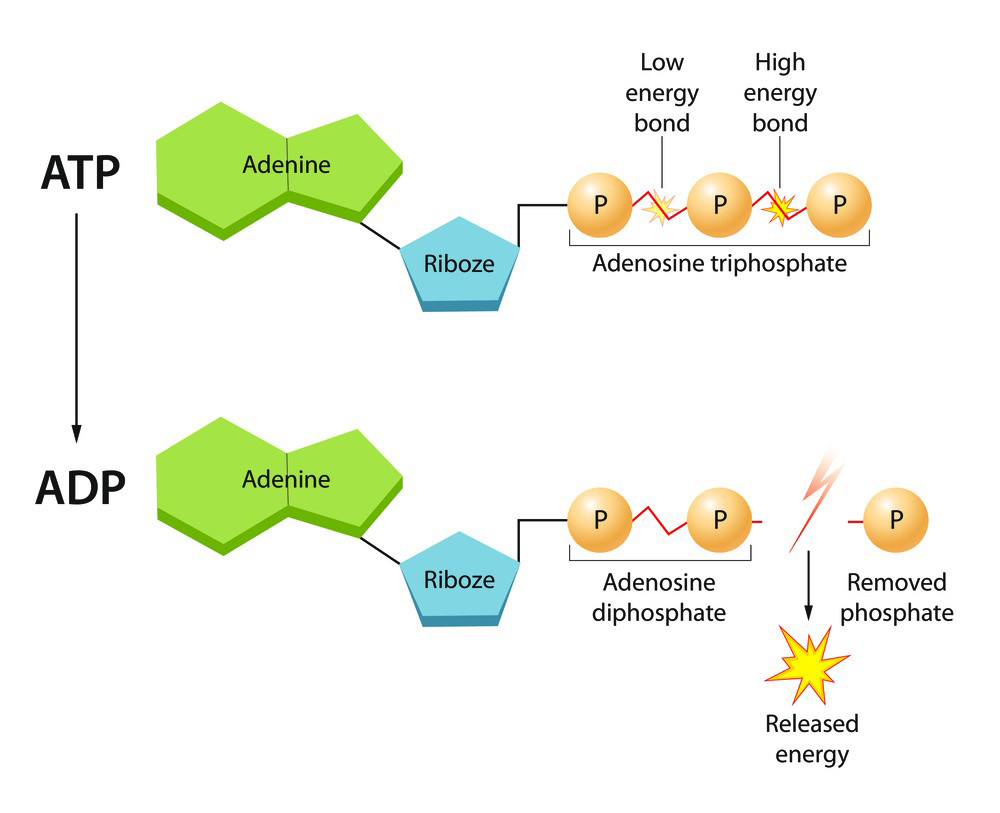
The structure of ATP consists of:
1.Adenosine: This is the base portion of the molecule and is composed of adenine, a nitrogenous base, and ribose, a five-carbon sugar.
2.Three Phosphate Groups: These are attached to the ribose sugar of adenosine. The phosphates are negatively charged and are bonded together in a chain.
The bonds between the phosphate groups are high-energy bonds, and when one of these bonds is broken (hydrolyzed), a significant amount of energy is released. This energy release powers various cellular processes, such as muscle contractions, active transport of molecules, synthesis of macromolecules, and many other essential biological activities.
The ATP molecule is constantly being synthesized and hydrolyzed in living organisms to maintain the energy needs of cellular processes. It acts as an immediate source of energy within cells, making it a crucial molecule for the functioning of all living organisms.
Pharmacological Effects of Adenosine Triphosphate
Adenosine Triphosphate (ATP) is a critical molecule that serves as the primary energy carrier in living organisms. While it is primarily known for its role in cellular energy metabolism, ATP also has important pharmacological effects on various physiological processes. Here are some of the key pharmacological effects of ATP:
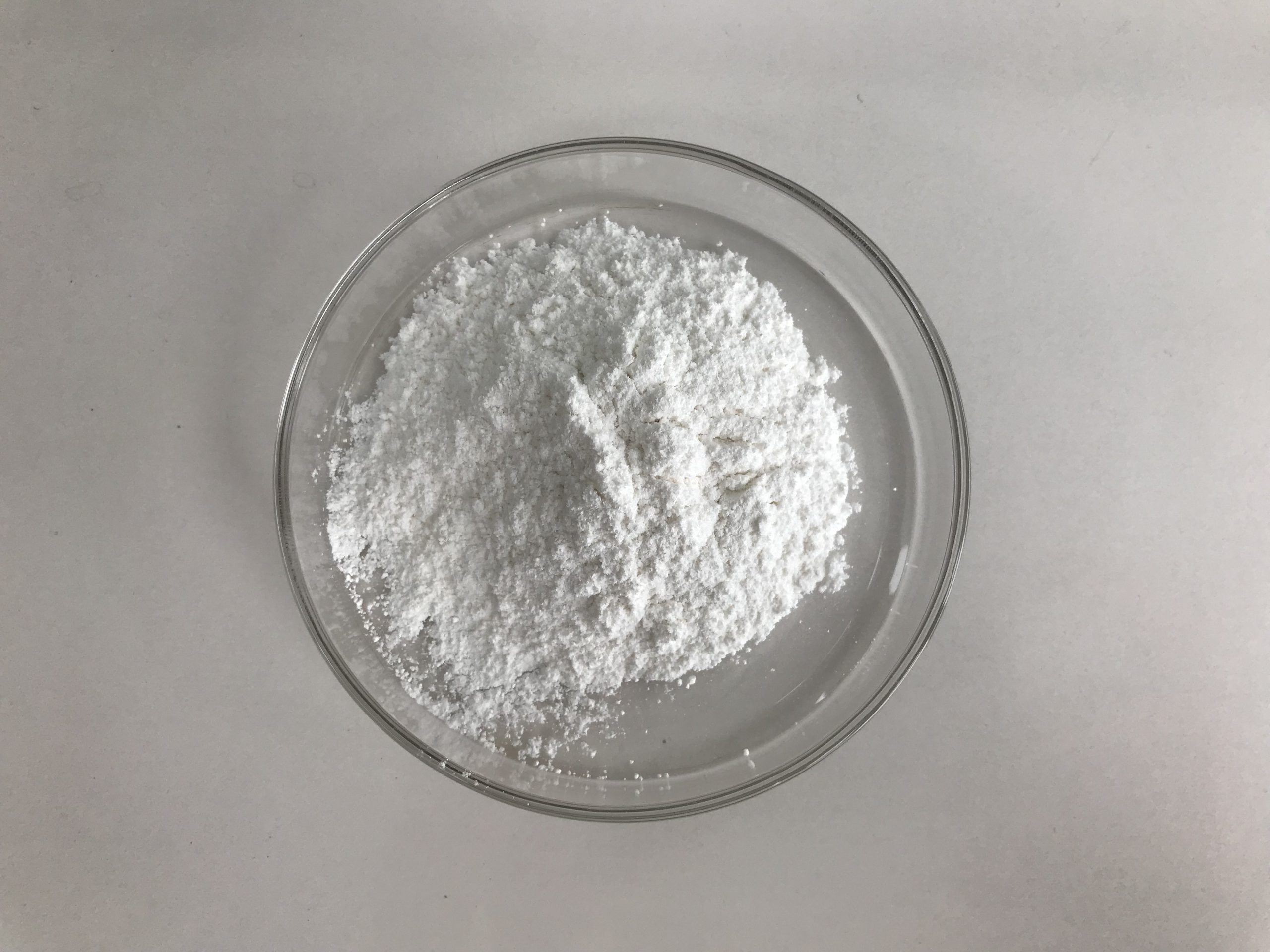
1.Energy Production: ATP is involved in cellular energy transfer and storage. It serves as a high-energy phosphate bond that can be rapidly hydrolyzed to release energy. This energy is used to fuel numerous cellular processes, including muscle contraction, nerve impulse transmission, and active transport of molecules across cell membranes.
2.Neurotransmission: ATP acts as a co-transmitter alongside classical neurotransmitters (e.g., acetylcholine, norepinephrine) in the nervous system. It is released from neurons and acts on purinergic receptors (P1 and P2 receptors) found on both pre- and post-synaptic membranes. ATP-mediated signaling is involved in synaptic transmission, neuromodulation, and plasticity.
3.Vasodilation: ATP can cause vasodilation (widening of blood vessels) through its action on P2Y receptors found on endothelial cells and smooth muscle cells of blood vessels. This effect helps regulate blood flow and blood pressure.
4.Pain Sensation: ATP contributes to pain sensation by activating purinergic receptors in sensory neurons. This mechanism is implicated in inflammatory pain and nociceptive signaling.
5.Immune Modulation: ATP plays a role in immune response regulation. It acts as a danger-associated molecular pattern (DAMP) when released from damaged or dying cells, activating the immune system and promoting inflammation. Extracellular ATP can also influence immune cell function through purinergic receptors.
6.Platelet Aggregation: ATP contributes to platelet aggregation, an essential step in the formation of blood clots. It activates platelet purinergic receptors, which trigger the release of platelet-activating substances.
7.Gastrointestinal Function: ATP participates in gastrointestinal motility and secretion regulation. It acts on enteric nerves and smooth muscle cells in the gut, influencing processes like peristalsis and digestive enzyme secretion.
8.Cardiac Function: ATP is involved in regulating cardiac contractility and heart rate through its actions on purinergic receptors in the heart tissue.
9.Respiratory Function: ATP plays a role in respiratory regulation by modulating the activity of respiratory centers in the brainstem.
Due to its central role in cellular energy metabolism and diverse physiological effects, ATP and its analogs have been studied for potential therapeutic applications in various conditions, such as cardiovascular diseases, pain management, and neurodegenerative disorders. However, the clinical use of ATP and its analogs is limited due to their rapid degradation in the body and challenges associated with their delivery. As a result, research is ongoing to explore potential therapeutic applications and targeted delivery mechanisms for ATP-based treatments.